What are EMFs? A rational, skeptical, curious person’s guide
When most people hear the term “EMFs,” they jump straight to “bullshit.” It sounds like a conspiracy theory, complete with literal tin foil hats.
But what are EMFs, really?
We’re well-trained that EMFs are something only crazy people talk about. That those people say 5G towers and Bluetooth devices are killing all of us. Or Bill Gates is installing microchips into people’s heads. Or whatever.
But interestingly, if most people are asked to define what an EMF actually is or what exactly they do and why they’re safe… they can’t.
That’s what this article is about. It’s a rational, skeptical, curious person’s breakdown of what EMFs are, really, from the ground up. This all came together because I realized I couldn’t explain why EMFs are safe, or — embarrassingly — what they even are and how they work.
And it turns out that EMFs are pretty interesting, and we interact with them all the time. They’ve just never been explained to us in a way that makes sense.
That’s what this article is here for. Along the way, we’ll revisit a bunch of scientific concepts that I’m willing to bet you’ve heard before — maybe back in middle or high school — but never quite got (and we’ll fix that).
Let’s dive in.
What are EMFs?
So, what actually are EMFs?
EMF stands for Electromagnetic Field. We’re going to build our understanding of them starting with something simple: visible light. And we’ll use that as the jumping-off point to learn about everything from radio waves to x-rays, and even (gasp) the dreaded “5G.”
(Spoiler alert: they’re all — including visible light — versions of the same fundamental force.)
Visible light is everywhere. it’s what allows us to see. It comes in a range of colors, familiar to any elementary schooler: from red to orange to yellow to green, blue, indigo, and violetIsaac Newton — the same brilliant scientist who outlined a theory of gravity after an apple apocryphally bounced off his head — listed off those seven colors in his 1672 letter “A New Theory of Light and Colors.” And they’ve lived on ever since. — and everything in between. Those are the colors of the rainbow (I still remember learning the mnemonic ROY G. BIV when I was a kid).
When the entire spectrum of colors are present, it looks like white light. And it turns out that if you pass white light through a prism, you can break it back into its component parts (remember Pink Floyd’s “The Dark Side of the Moon” album art?).
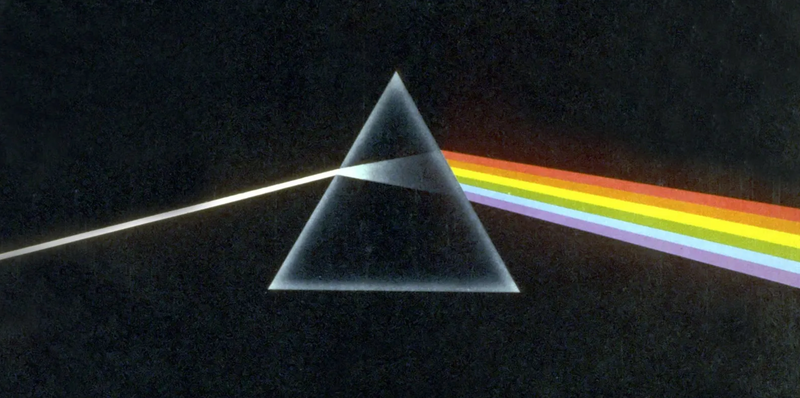
I mentioned that red, orange, yellow, green, blue, indigo, and violet (and everything in between) are also the colors of a rainbow — and rainbows themselves are created in much the same way. Pure white light from the sun shines through many tiny raindrops in the atmosphere, and those raindrops function as mini-prisms, breaking up the white sunlight into its rainbow-colored constituents.
So white light is made up of the entire spectrum of colors. But have you ever wondered why those colors — whether refracted through a Pink Floyd prism or an atmospheric raindrop — always show up in the same order? You never get a rainbow that goes from red to blue to yellow to purple.
To understand this, we’re first going to take a quick step back.
All light has a property called its “wavelength.” Think about tossing a rock into a pond and seeing the wave rippling out. The distance from one ripples’s peak to the next is the “wavelength” of that wave. And light is the same way — it goes up and down in intensity constantly, and the distance between each of these peaks is the wavelength.
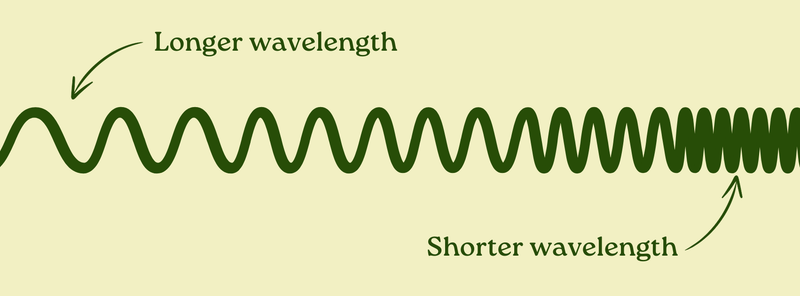
When we examine light, we find that the wavelength of the light corresponds to its color. All visible light has a wavelength between roughly 740 nanometers (about 1/100th the width of a human hair) at the long end, and 380 nanometers at the short end. We can arrange them along a spectrum like this, from longest to shortest:
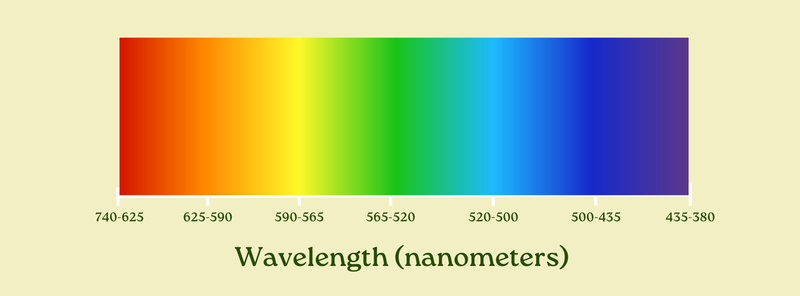
Here’s a cool little demo that illustrates this. Enter a different wavelength and you’ll see the color change.
Light waves with a wavelength of 740 nanometers? Very red. 490nm? Cyan. 585? Yellow, but by 600 it starts to get pretty orange. 395 is a royal purple, and 440 a bright blue.
Our eyes perceive different colors by distinguishing between the varied wavelengths of the light coming in — if you’re seeing red, it’s because 700-something nanometer light is in your face. A blue sky? You’re getting more light in the high-400s.
Importantly, our visual system can only see light between 740 and 380 nanometers.
Prisms or raindrops split white light up into its constituent colors because when the white light comes into the prism, it begins to bend (see the Pink Floyd cover). And, because each of these colorful components of the white light has a different wavelength, they bend at different speeds! The violet with its shorter wavelength is able to make a much faster turn than the red with its longer one.
Roses are red,
Violets are blue,
Long waves bend gently,
Short ones twist through.
So you end up with a perfect splaying-out of the colors — the longest red waves bending the least, and then the oranges, yellows, greens, blues, indigos, and last, the violets turning the most.
You may be wondering when we’re going to get to EMFs. We’re almost ready to talk about cell signals and Bluetooth and Wi-Fi and the like, but also: visible light itself is an EMF (yes, really). Back to that in just a moment.
What’s beyond light?
We now understand that light is a wave, and that its wavelength determines its color. And we understand that there’s a spectrum from long-wavelength red all the way through short-wavelength violet.
But that leads to an interesting question: what would happen if we took some of that short-wavelength violet light and made the wavelength just a little bit shorter? Said another way: what exists here?
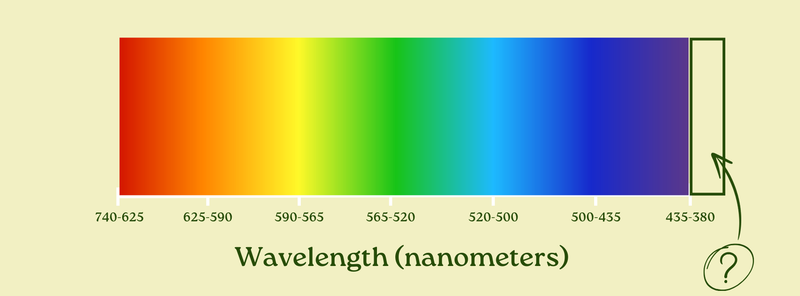
We’ve already said that our visual system can only see between 380 and 740 nanometer light. So if there was light at, say, 375 nanometers… it wouldn’t be visible. The wavelength would be smaller than the lower bound of our visual system.
At the same time, it seems possible to have light with a wavelength shorter than 380 nanometers. In fact, it certainly is. But again: it’s not visible to our eyes.
What if it could be seen? Use your imagination, projecting beyond that color spectrum. What would it look like? I think it would look like the violet-iest violet you can imagine… something so violet it’s like a mega-violet. Or a super-violet. Or maybe…
Ultra-violet?
That’s right. Ultraviolet light — or “UV” — is light that is just outside the visual spectrum next to violet. It’s still the same thing as the rest of the visible light, but we can’t see it.
It includes light from about 380 nanometers to about 10 nanometers, and it’s responsible for both the pain of sunburns and the hygiene of UV cleaning.
Enterprising readers may have jumped to the next conclusion as well: what’s on the other end?
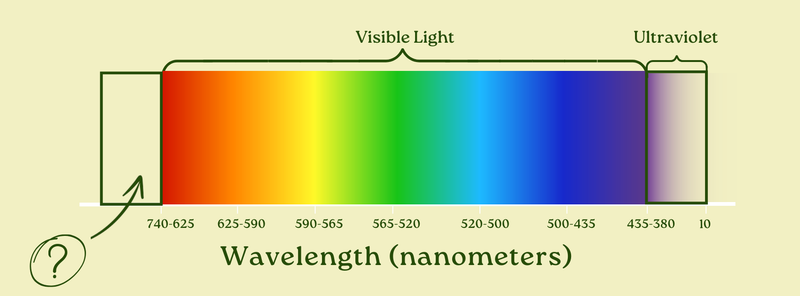
If you take red light and make it slightly shorter, you get orange. But if you make it slightly longer, you get…
(Any guesses?)
Infrared.
Infrared light — just like ultraviolet — is light that is just outside of our visual range. But instead of being shorter than violet, it’s longer than red. It goes from about 740 nanometers to about 1,000,000 nanometers.
Infrared light in different intensities is used for lots of things, from remote controls (ever seen that bulb on old remotes?), to thermal imaging like night-vision goggles, infrared saunas, and so much more.
Okay. Nice. So there’s visible light, and then there’s invisible light on either side of it. Infrared’s longer wavelength light, then red-orange-yellow-green-blue-indigo-violet visible light (from longest to shortest), and then ultraviolet light (even shorter).
But now, once again, some readers may be jumping ahead to the next question. What’s ultraviolet-ier than ultraviolet light? If you took the ultraviolet light with the shortest wavelengths, and you made it even a teeny bit shorter, what happens then?
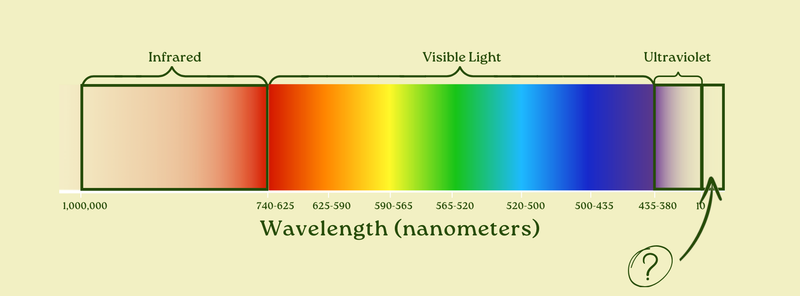
If you’re tempted to say you get überviolet light, then I applaud you (but you’re wrong). The actual answer?
X-rays. (Yes, like the ones you get at the dentist.)
Wait, what? We went from sunlight to x-rays just by changing the wavelength of the light?
Indeed. And what lies on the other side of infrared? Just a little longer than the longest, 1,000,000 nanometer infrared waves?
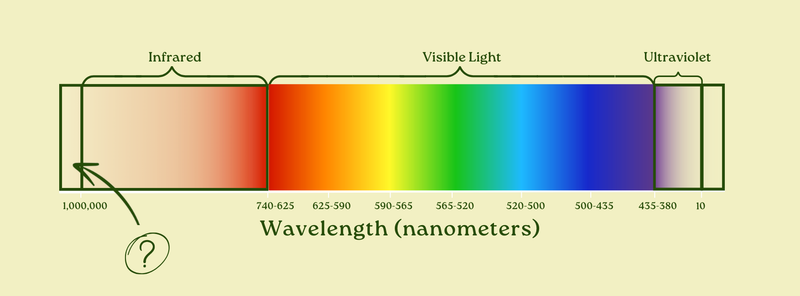
Millimeter waves, a.k.a. the waves used for 5G cell signals (yep), the full-body imaging systems that TSA uses, and more.
Here’s the punchline of everything we’ve been building up to here…
Electromagnetic Fields — EMFs — encompass a huge spectrum of waves. They’re all the same thing at a deep, fundamental level, differing only in their wavelengths (and, correspondingly, frequencies and energies, as we’ll get to shortly).
Much like a guitar’s strings are all the same fundamental thing (a string), but yet different in their length and thickness, EMFs are all the same thing (an electromagnetic wave), but different in their wavelength and frequency.
And much like plucking one string on a guitar creates a high note, and another a low note — one EMF can express itself as blue light to be seen by eyes; another an x-ray to image a body; another a 5G signal to transmit information; and another an infrared light to make you sweat in the sauna.
And, much like you can pluck a guitar string hard or soft, quickly or slowly, so too can EMFs be of different intensities and transmissions patterns.
We’ll get back to all that. But first, let’s reveal the fuller electromagnetic spectrum:
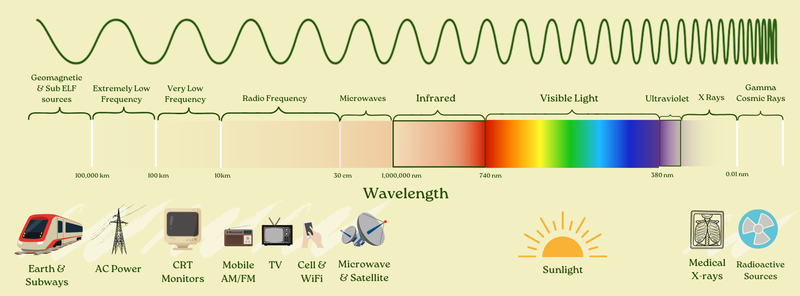
It goes from the natural electromagnetic field of the Earth to AC power (what we use to power our homes) to radio to TV, cell phones, microwaves, satellites, infrared, visible light, ultraviolet light, x-rays, and gamma rays — and lots in between.
If you walk away from this article with nothing else, let it be this:
All of these are EMFs.
And EMFs, it turns out, do a whole lot. Some of it is really, really good — like letting us see! Or allowing us to communicate over distance! Or powering our houses! Or helping us diagnose broken bones!
But, as with most any technology out there in the world, in addition to being great, EMFs can also be bad, depending on the dose and the exposure.
X-rays let us see broken bones — but in too high a dose can cause cancer.
UV light exposure helps us produce critical vitamin D — but can give us sunburns.
Power lines deliver us electricity — but can shock us.
Microwaves can heat our food — but without proper shielding can burn us.
Gamma rays are used for targeted cancer treatment — but can also cause cancer themselves.
All that to say: same as every other technology, we need to be mindful about EMF. We’re careful with knives (despite them being life-changing for food prep), the internet (despite it changing the fabric of human civilization), and the wheeled vehicles (despite them shifting the trajectory of humanity). EMFs are no different.
You’re probably reading this article in the first place because you want to separate fact from fiction — and be able to dispel bullshit — about “EMF danger.” And so a general “be mindful, like with any other technology” isn’t terribly useful. We’ll get back to a rational look at the risk profile of EMFs in a minute, but in order to do so rigorously, we first need to cover just a little more scientific background.
A little more EMF science
First: frequency and amplitude.
We talked sometime earlier about the ripples that come from a rock tossed into a pond. We used that to discuss the wavelength — the length of each of the ripples, from peak to peak. There’s another attribute we could talk about, and that’s the “frequency.”
Frequency is simple: it is how many waves pass a given point each second. If you stared at some part of the pond and just counted up how many waves passed through that area in a period of time, you’d have the frequency.
Frequencies of electromagnetic radiation are expressed in the number of waves “per second,” which is called “Hertz” (or Hz for short). So if you see something like “10Hz,” that means the wave is passing a given point 10 times per second. Pretty simple.
Now, any wave’s speed is the wavelength times the frequency. This is pretty simple when you think about it.
By analogy: let’s imagine you’re running, and the length of your stride is 0.7 meters (“stridelength,” or “wavelength”). And let’s say you’re running at 2.5 strides per second (stride “frequency”).
Your speed (a.k.a. distance covered over time, like “miles per hour”) is going to be the length of your stride times the number of strides per second:
0.7 meters * 2.5 per second = 1.75 meters per second
So you’re running at 1.75 meters per second, thanks to a stride length of 0.7 meters and a stride frequency of 2.5 Hz (a.k.a “per second”).
This can be expressed as an equation (don’t worry, we’re not going to get too math-y here):
wavelength * frequency = speed
And if you remember some basic algebra, we can look at that equation in a different way:
frequency = speed / wavelength
If you know the speed and the wavelength of a wave, you can figure out its frequency. Easy.
Now, let’s talk about red light. Wavelength: 740 nanometers, like we talked about earlier. Do you have any idea what the speed of that light is?
It’s — perhaps not shockingly — just the classic speed of light. (This happens to be 299,792,458,000,000,000 nanometers per second). As you may remember, the speed of light is constantFor the sake of simplicity, we’re assuming all of this in a vacuum. The math gets a little more complicated if we don’t, but still holds! — it’s always that same number.
And so, we can figure out the frequency of red light: it’s just that big number for the speed of light divided by the red light’s wavelength.
299,792,458,000,000,000 nm/s divided by 740 nm = 405,124,943,243,243 Hz
Or, a little more simply: around 405 tera-hertz.
You could do the same math for violet light, at 380 nm, and you’d get 788 terahertz as its frequency.
What we’re realizing here is something very interesting: all light moves at the same speed (the speed of light). And that means that, since wavelength times frequency equals speed, if we know the wavelengths of all those light colors (we do), we can also figure out all their frequencies.
And so, the higher the wavelength, the lower the frequency; the lower the wavelength, the higher the frequency.
We already knew that red light has a longer wavelength than violet light, and there’s a whole spectrum in between. We now also know that violet light has a higher frequency than red light, and there’s a whole spectrum in between.
And, of course: just as before, all of this applies to every other part of the electromagnetic spectrum.
Yes: all of these things, from electrical power to radio waves to microwaves to infrared, visible light, ultraviolet, x-rays, gamma rays, and everything in between all travel at the speed of light. Once again: they’re all pretty much the same thing.
So we can extend our fuller diagram as well, showing both the wavelength and the frequency.
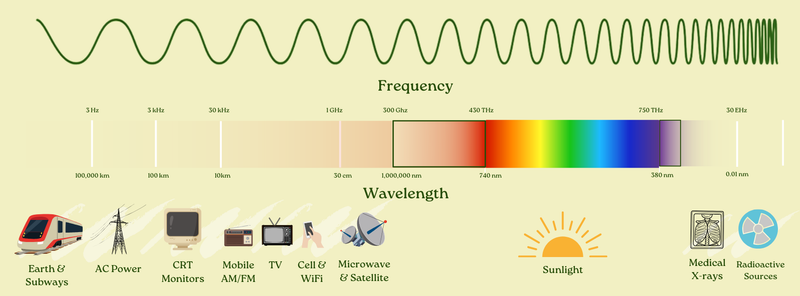
The resonant components of the Earth’s natural electromagnetic field (the Schumann resonances, which are generated by lightning between the Earth’s surface and the ionosphere) are really slow with really long waves: starting at around a 7.83Hz frequency with a 38,000 kilometer wavelength.
And on the other end, gamma rays — often seen out in the cosmos — are really fast with really short waves.
To give you a sense of scale: the difference between the wavelength of the Schumann resonances (around 38,000,000 meters) and the wavelength of gamma rays (around 0.000000000003 meters) is about 13 trillion trillion times. That ratio is even larger than the ratio between the size of a single atom and the entire Earth.
The frequency of those Schumann resonances (7.83Hz) is almost in range of you being able to count it yourself — 8ish “ticks” per second. But the frequency of gamma rays? They oscillate 10 quintillion times per second (10,000,000,000,000,000,000 times per second)!
And there’s a whole spectrum in between. I find it pretty incredible that these are all the same fundamental force, and yet are so, so different.
Last up: amplitude. We’ve been talking about these “waves” — and even visualizing them — but we haven’t talked about the height of the wave (that’s the amplitude).
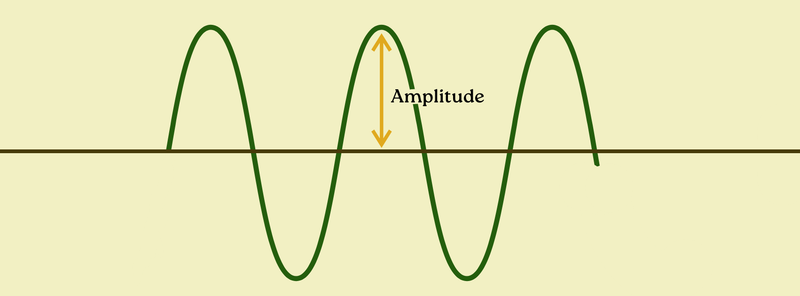
We now know that red light is always around 740 nm in wavelength and 405 terahertz in frequency, but that same red light can be more or less bright, of course. Same with anything else on the electromagnetic spectrum. The “amplitude” is the strength of the field.
And again, this is just like waves in water — we can look at their wavelength (distance from peak to peak), their frequency (number of waves that pass per second), and their amplitude (height of the wave).
And this is now a great jumping-off point for talking about the health impact (or not) of EMFs.
Ionizing EMFs
There are categorically two ways that any EMF could in theory cause harm to a biological system (like our bodies): through ionizing effects or through non-ionizing effects.
I’m going to briefly cover ionizing radiation first, but then we’ll focus on non-ionizing effects — because that is where the current controversy lies, and where we need to be better equipped to understand.
There’s some irony here, because to understand ionizing radiation (the completely non-controversial type of EMF harm), we actually need to take a quick detour through a quantum view of an electromagnetic field (which sounds even more like bullshit to most people). If you’d rather skip that and get to the controversial non-ionizing stuff, click here.
I’m going to speed through this in bullets with reference reading, in the interest of keeping this already-long article as brief as possible and avoiding a full Quantum Mechanics 101 syllabus. Here’s the summary:
- all light — which means all electromagnetic fields — can exhibit both particle and wave properties (see: Wave-particle duality and the Double-slit experiment)
- we’ve been talking all about the classical mechanical view on electromagnetic fields, which discusses these fields as waves rather than their particle nature
- but if we take the quantum view (rather than classical), electromagnetic fields are intermediated by photons, the elementary quantum of EMFs. This is a bit like viewing an ocean wave as a collection of water molecules rather than as a big wave (although don’t take that too far — photons are massless and not particles in the sense most people think of them)
- as described by the Planck relation, the higher frequency a wave is, the higher the photon energy of its “constituent” photons
- this means that, regardless of the overall amplitude/intensity of the whole wave itself, the photons “comprising” a high-frequency wave like a gamma ray are super high energy, and the photons “comprising” a low-frequency wave like a radio wave are much lower energy
- in fact: the amplitude of the wave doesn’t matter at all for the energy of the individual photons within it (but it does matter for the intensity of the overall wave)
And — here’s the rub — if a photon is high enough energy (meaning the wave it is a part of is a high-enough frequency wave), it can ionize atoms and molecules, which is as scary as it sounds.
Ionizing means that when a super high energy photon (from a high-frequency wave) “hits” something, it can knock out electrons from that atom or molecule, creating chemical changes and potentially severe biological damage. This includes various types of cell and DNA damage, leading to mutations and cancer.
So the reason x-rays can be scary — or the gamma rays coming off radioactive waste — is because those are really, really high-frequency waves. Really, really high-frequency waves means really, really high energy photons. And really, really high energy photons means potential cellular or DNA damage if they hit you.
Yikes.
Here’s the comforting part, though: most EMFs are what’s called “non-ionizing.” This means that their frequencies are low enough (and correspondingly, wavelengths long enough) that their photons simply don’t have enough energy to do ionizing damage.
Scientists draw the ionizing vs. non-ionizing cutoff at about 2.4 * 1015 Hz frequency, or 125 nm wavelength (although it depends on the exact type of matter it is interacting with). Waves at a lower frequency than that (or a higher wavelength) aren’t ionizing.
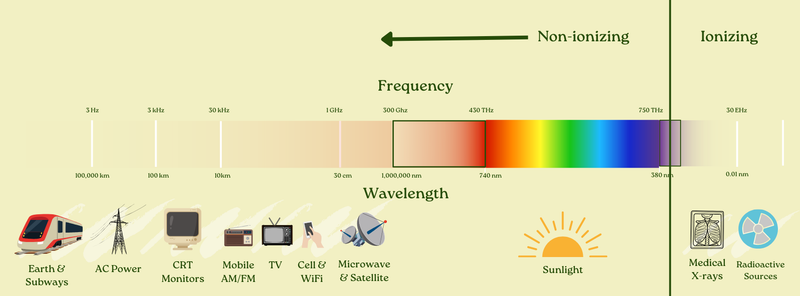
Ionizing waves include the very high-frequency end of ultraviolet light (part of UV-B and all of UV-C light) and everything above that — including x-rays and gamma rays.
So for those types of electromagnetic fields, everyone agrees: be careful. This is a bit part of why we’re careful with radioactive waste; why you get protection when getting an x-ray and the technician often leaves the room; and why certain extreme parts of the UV spectrum can cause health issues.
(Now, of course: the danger doesn’t mean they aren’t still useful sometimes. We use gamma rays to precisely kill cancer cells, x-rays to see through our bodies, and more.)
Here’s an imperfect but useful analogy: imagine you were in the ocean and waves were hitting you. But now, let’s imagine that you somehow heated up the water molecules in those waves a lot. It doesn’t matter how tall or short the waves are — at some point when the water molecules get hot enough, you’re going to have a problem. You could be facing the lowest-intensity physical waves, but if the water is boiling (read: the EMF photons have enough energy), you’ll suffer.
Electromagnetic radiation is much the same way. The heat of an ocean wave’s particles is somewhat analogous to the photon energy of the field’s photons. Thankfully, below a certain temperature, the heat component of a wave just won’t bother you — might even feel nice! And similarly, below a certain photon energy (and correspondingly below a certain frequency), an electromagnetic field just can’t ionize you.
But to what, then, can we analogize the other ways an ocean wave could hurt you?
Non-ionizing EMFs
Beyond our burning water example, I’d say there are two ways an ocean wave can hurt you: a really intense wave can hammer you physically, or a specifically-shaped wave can disrupt an otherwise finely-calibrated system (like by getting water in your eyes, or down your windpipe).
(And most of the time, playing in the waves is just fine!)
I think of non-ionizing radiation in the same way. Mostly fine and lovely. But if it can harm you, it does so through one of two ways: either a lot of intensity causing a “smack” (in science terms, in the case of EMFs this is what’s called “thermal effects” — we’ll get back to this), or a disruption of a system in your body (we’ll get back to this too).
Let’s look at an example of each of those types of non-ionizing radiation that could cause you harm.
Non-ionizing but thermal
In the 1940s, a American scientist named Percy Spencer stumbled upon the realization that electromagnetic waves could heat things up. The story goes that he was building magnetic equipment for the US World War II effort and a candy bar in his pocket melted thanks to the generated waves. He (via his employer, Raytheon) went on to patent the microwave oven in 1945, although it took a couple more decades before an affordable consumer version was produced.
Microwave ovens use, unsurprisingly, micro-waves — electromagnetic radiation with wavelengths around 12.5cm and frequencies around 2.4Ghz (that’s giga-Hertz, and could also be written as 2,400,000,000 Hertz). They do so because this type of radiation is extremely effective for heating up water molecules.
It’s pretty obvious that if you put your body in a microwave oven, it would not be a pretty picture. They are extremely effective at heating up organic (water-containing) tissue, like our bodies.
Clearly, these waves could cause you harm — imagine your insides heating up the same way a piece of pizza does. That’s because the waves inside the ovens are at such high intensity — and that’s why microwaves are shielded.
What’s the shielding? Well, most walls of the microwave are metal, which does a good job blocking this type of electromagnetic radiation. And have you ever looked at the front of the oven? It typically has a metal mesh-looking material over it (encased in glass).
How the mesh works is pretty cool: electromagnetic waves generally don’t easily pass through metal/conductive holes that are much smaller than their wavelength. The microwaves are around 12.5 centimeters in wavelength, and the mesh holes are usually around 0.1 centimeter. So the mesh “catches” the microwaves pretty well, and saves you from having your insides absolutely roasted along with that slice of pizza when you’re next to the microwave (although a surprising amount of radiation still leaks out!).
Anyway, all this to say: non-ionizing EMFs — like 2.4Ghz waves from microwaves — can cause damage to organic tissue (like our bodies) at sufficient intensities. If the amplitude of the waves are high enough, the intensity gets big enough, and that can cause these “thermal effects” — where tissue gets heated up, causing damage.
Think of this like the equivalent of a really tall wave in the ocean. If a wave is big enough, when it hits you, it can physically hurt. Electromagnetic waves like microwaves are similar in this sense — with enough intensity, they can heat you up and cause damage (again, imagine being inside a microwave oven).
Over the course of history, our understanding of the potential harm of electromagnetic fields has evolved. For a long time — starting in the 1800s when all this was starting to be discovered — our broad scientific understanding was that the only way an electromagnetic field could directly hurt you was if it was ionizing. We knew x-rays and gamma rays and such could be harmful. But we established that everything else below that range — anything starting in the ultraviolet range or below — was safe.
But over time, we started to realize that wasn’t quite true (in part because we hadn’t built really powerful & intense electromagnetic systems yet). It took until the mid-1900s and the creation of the microwave for us to realize that there was a non-ionizing way that EMFs could cause harm: those thermal effects.
Even then, it really took until the 1980s for this to get recognized from a regulatory and mainstream perspective. Until then, the consensus was “ionizing = bad; non-ionizing = fine.” But by the 1980s, we added another category of harm: ionizing was definitely still bad, thermal effects could be bad, but everything else was still definitely fine.
And weirdly enough, that seems to be the general popular stance even today: if an EMF isn’t ionizing or causing thermal effects, it must definitely be safe.
But I, honestly — after doing all this research into this — don’t really understand how anyone who has thought deeply about the subject can believe that. And it goes back to one of the categories I mentioned above: how a specifically-shaped ocean wave can disrupt something in your body even if the wave isn’t boiling hot or super intense (like getting water down your windpipe).
Non-ionizing but disruptive
Here’s a dead-simple example: blue light.
Blue light is, as we’ve been talking about, an EMF. It’s an electromagnetic wave with a wavelength around 440nm and a frequency around 750 THz — well below the ionizing threshold.
But blue light is known to be harmful for our sleep quality and Circadian rhythm! That’s why using your computer or phone late at night can make it hard to sleep. This is well-established and I’ve never heard anyone well-informed argue that blue light has no negative effectsI’ll write more about this in the future, but for now, some sources: 1, 2, 3, 4..
It’s bad for you, but it’s not because it is ionizing radiation, nor is it high enough intensity to have thermal effects. It disrupts a very finely-attuned system in your body: your visual system, which has evolved expecting blue light only in certain situations (namely: when the sun is up).
So, clearly: non-ionizing and non-thermal EMFs can have negative effects. I’m certainly not saying they all do. But I’m simply pointing out that this public view that EMFs are safe if they don’t heat you up or ionize you may not be broadly true.
So what?
So now we understand that EMFs can cause damage in one of three ways:
- High-enough frequency EMFs (the high end of UV and above) are ionizing, causing cellular and DNA damage
- Certain EMFs at high enough intensity can cause thermal effects, heating up tissue and causing damage
- EMFs could hurt you in other ways, by disrupting systems in your body (in some sense, this is a catch-all for other categories)
So, again, like an ocean wave: it could be heated up and burn you regardless of intensity (case #1), it could be really tall and intense and physically hurt you (case #2), or it could hit you in just the right way — imagine a tall, skinny, not-intense wave splashing into your face — to hurt you another way (case #3).
But I’ll advance this even a step further before getting to “so what.” Because there are at least a few other factors that could impact if a wave hurts you, beyond the characteristics of the type of wave (wavelength & frequency) and the intensity (amplitude).
First: the duration or pattern of exposure to the wave. Sure, one medium-intensity ocean wave might not hurt you. But if you stood and got smacked identical waves for 8 hours? Might start to hurt. The cumulative “damage” from the waves could be real. The same could be true for the “system disruption” case — maybe one wave is fine, but after an hour of them, you’ve got salt water in your eyes and it really burns.
And second: your individual sensitivity. Maybe a certain ocean wave doesn’t have any negative impact on a 6 foot tall, 200 pound person — but that same wave could send a baby flying. Or it could even knock over a taller, thinner person. Or it could take out someone of the same size, but who has worse balance. Or maybe someone has open wounds on their body and regardless of their size, the wave really hurts them.
And of course, electromagnetic waves are much the same.
All this to say: to properly evaluate the impact of an EMF on a biological system (like our bodies), you need to think about at least four things:
- the characteristics of the wave type (wavelength, frequency)
- the intensity of the wave (amplitude)
- the duration or pattern of exposure
- the individual sensitivity
This is — by the way — not such a crazy thing to do. Just think about sunlight! It’s an EMF. And when you’re assessing the potential for sun damage, you’re thinking about:
- What time of day is it? (Early morning & evening means a lower proportion of high-frequency UV light, thus lower risk for sunburns relative to midday. This is you — without even knowing it — thinking about the characteristics of the wave type, like frequency)
- How bright is it given where I am and the time of year? (This is you considering the intensity of the wave)
- How long am I going to stay out? Have I already been out a bunch today? (This is you looking at the duration or pattern of exposure — more exposure means more risk)
- How sensitive am I to the sun? (We all know we have a different individual sensitivity, and take that into account)
When you look at it that way… maybe not so unreasonable for us to take a nuanced view on other types of EMFs, too.
Conclusion & what’s next
We just got through a lot: what EMFs fundamentally are, some of the common types of EMFs, what a few of their key attributes are, and then the ways they could harm you and how you can think about the harm.
To sum it all up:
Electromagnetic fields are waves, including everything from the Earth’s electromagnetic field to radio waves to WiFi to TSA body scanner waves to visible light, ultraviolet light, x-rays, and much more. It’s a full spectrum, each defined by their wavelengths and frequencies (which are inversely correlated with each other).
These waves aren’t inherently good or bad, any more than gravity is good or bad. They provide so much benefit to humanity, but they can also be harmful.
When they’re harmful, it’s in one of three ways:
- The highest-frequency waves are ionizing, which means they can cause direct cellular and DNA damage and are clearly carcinogenic — like x-rays and gamma rays
- Certain waves at a high enough intensity (or amplitude) can cause thermal effects, heating up organic matter — like microwave ovens do
- Some waves could disrupt our other biological systems without being ionizing or having thermal effects — like blue light affecting our sleep and Circadian rhythm
And in order to evaluate that harm, we need to look at four factors:
- The wave characteristics — its frequency and wavelength
- The intensity of the wave — its amplitude
- The duration and pattern of exposure
- The individual’s sensitivity or characteristics
I hope this has taught you a thing or two about EMFs — I learned a lot while researching it, too. But you may be left here with more questions than answers. We’ve developed the foundation for being able to talk about if and when EMFs are harmful, but we haven’t actually done that evaluation on any of them.
And the questions you might be asking now are: these 5G towers going up — are they bad or is that all fiction? What about big power lines? And is having our Bluetooth, WiFi, and cell-service-enabled phones in our pocket a problem?
After doing a whole lot of research, it’s clear to me that the health impacts of EMFs are severely underrated by the general public and media.
Nobody is disputing that x-rays or gamma rays are harmful. But the popular public narrative is that everything else is fine.
However, there’s significant, compelling evidence that radiofrequency EMFs — like those from WiFi, Bluetooth, and cell service — and even ELF (extremely low-frequency) EMFs can be harmful. And that flies in the face of the public narrative.
See, for example, Dr. Henry Lai’s review of about 2,500 studies on radiofrequency and extremely low frequency EMFs conducted between 1990 and 2024. He found that 70-90% of the studies reported significant effects across oxidative stress, genetic effects, neurological impact, and reproductive/development issues.
I often hear people say “there’s zero evidence that those EMFs are harmful!” But I find it hard to look at Dr. Lai’s review of 2,500 studies and come to that conclusion.
Or look at the United States’ National Toxicology Program’s 2018 report. After a 10-year, $25MM study on radiofrequency radiation, they found clear evidence of an association with tumors in the hearts of male rats, and some evidence of an association with tumors in the brains and adrenal glands of male rats. And this was from 2G and 3G radiation — not 4G or 5G, which are both higher frequency.
This was the first public US government study into the health impacts of radiofrequency radiation since the 1990s (and clearly wireless technology and exposure has changed a lot since that time). The FDA asked the NTP to conduct this study in May 1999 (19 years before it was published), saying in part:
Currently cellular phones and other wireless communication devices are required to meet the radio frequency radiation (RFR) exposure guidelines of the Federal Communications Commission (FCC), which were most recently revised in August 1996. The existing exposure guidelines are based on protection from acute injury from thermal effects of RFR exposure, and may not be protective against any non-thermal effects of chronic exposures
If you want to learn more about the flawed assumption at the heart of US radiofrequency radiation regulation, check out this followup post I wrote.
I’ll publish more in the future about my evaluations of individual types of electromagnetic radiation based on the research, and what you can or should do about them. But, in the meantime, below are some precautionary steps I have personally taken.
These are mostly easy and low-effort, and given the uncertainty that exists around this topic, it feels worthwhile to take these basic steps:
- I don’t hold my phone up to my head — earbuds or speakerphone only
- I try not to keep my phone in my pocket — when I sit down, put it on the table
- I use wired headphones instead of wireless, and turn Bluetooth off
- I use wired peripherals for my computer instead of Bluetooth
- I turn WiFi off at least at night (actually, I’ve hardwired my house with Ethernet so I don’t need WiFi at all)
Because of the nature of electromagnetic fields, even a small amount of distance can create a huge reduction in exposure (thanks to the inverse-square law).
Will taking those actions make a meaningful difference in my life and health? I do not know. But they are easy to do, and I think worthwhile given the evidence I have seen.
This can be a fraught topic, but I’m looking forward to writing more on it. If you’re interested in discussing or have any questions (or any corrections!), please don’t hesitate to reach out.
Looking for more to read?
Want to hear about new essays? Subscribe to my roughly-monthly newsletter recapping my recent writing and things I'm enjoying:
And I'd love to hear from you directly: andy@andybromberg.com